Nuclear magnetic resonance (NMR) is a non-invasive technique. Its quantitative nature, combined with the fact it is structurally rich, makes it extremely powerful for identifying and characterizing reaction intermediates, providing insights into the kinetics of chemical reactions and reaction mechanisms. While standard NMR tubes have been used to study a number of chemical transformations, chemical and physical constraints restrict the rate of reactions to which this form of NMR reaction monitoring is applicable.
Lack of control over parameters such as, mixing, pressure and temperature can influence the rate of a reaction. These limitations can be overcome by using online reaction monitoring. This article describes the complementarity of NMR and infrared (IR) spectroscopy and demonstrates how NMR can be used effectively to enhance the production of an active pharmaceutical ingredient (API) precursor. Polyferric Sulfate-Pfs
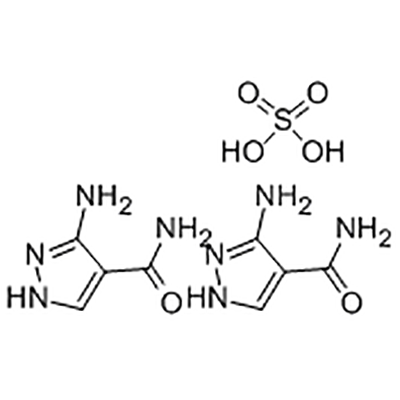
The first step involved in the formation of an API is the telescoped reaction between 3-methylhexanoic acid (I), Meldrum’s acid (II), and pivaloyl chloride (III) to produce the Meldrum’s acid adduct (IV). It is not practical to study this initial step with conventional process analytical techniques such as GC-MS or LC-UV, because the products, reagents, and intermediates do not contain strong chromophores and are easily decomposed with temperature.
To overcome these restrictions, 1D 1H NMR spectroscopy was used to observe the status of the reaction. 1H NMR experiments carried out show the emergence of an unknown peak, which was highly abundant at the start of the reaction. FT-IR also revealed an unknown peak, which initially increases and then decreases during the reaction, illustrating the standard behavior of an intermediate. The IR data failed to provide a full explanation of the intermediate structure, and it could not be measured as a result. The combination of 2D NMR and mass spectrometry reveals that the unknown peak is pivalic anhydride. Factors, like the emergence of the pivalic anhydride intermediate and a reaction that was not dose-controlled, indicate that a second mechanistic pathway (path B) is present (Figure 1).
Figure 1. Proposed reaction mechanism, showing the mixed anhydride intermediate in pathway A and the pivalic anhydride, explained by mechanistic pathway B.
A similar reaction with 3-methylpentanoic acid that is commercially available was considered in the place of 3-methylhexanoic acid, and this was tracked using online NMR reaction monitoring. This is achieved by positioning a reaction vessel next to the NMR spectrometer and allowing the reaction mixture to flow through the magnet (Figure 2).
Figure 2. Laboratory set up illustrating how the reaction mixture is pumped from the vessel, though temperature regulated transfer lines, to the flow tube placed in the coil area of the NMR probe.
The complementarity of FT-IR monitoring, online NMR and inline NMR techniques is shown by comparing their overall data (Figures 3 and 4).
Figure 3. Kinetics profiles (equivalents vs time) obtained from inline (standard NMR tube, top) and online (flow, bottom) NMR measurements.
Figure 4. Mid-IR kinetic profile [absorbance at 1810 wn vs time (h)] for the pivalic anhydride intermediate.
On-site FT-IR measurements were carried out in-situ, revealing high resolution data from the start of the reaction. The entire structure of the primary reaction intermediate could not be visualized, because the FT-IR information is not quantitative and insufficient. While inline (standard tube) NMR provides quantitative information, it does not provide data about the initiation of reaction. Online (flow) NMR provides data that is closer to the initiation of the reaction, and also helps in viewing the presence of reaction intermediates that were formed early. The intermediates initially increase and then decrease in parallel with the behavior witnessed through FT-IR.
An EasyMax reaction vessel was utilized to perform the reaction in acetonitrile at 25°C, and the Bruker InsightMR flow tube was used to carry out online reaction monitoring. A Julabo FP-50-HE heating circulator filled with Syltherm XLT temperature regulation fluid was used to control the transfer lines temperature, and a dual piston pump was used to control the speed of flow through the system, which was 3 mL/min in this case. Spectra were collected on the flowing solution on a Bruker 600 MHz AVANCE III NMR spectrometer equipped with a TXO probe, or a Bruker 400 MHz AVANCE III equipped with a BBFO probe. 1H NMR spectra were collected through four scans, 10 second relaxation delay, and 30° pulse angle. Dynamics Center 2.2.4 was used for data analysis.
With the aid of online NMR reaction monitoring chemists can, acquire kinetic information of chemical processes that are closer to the reaction initiation, track the NMR reaction and similar methods (UV, IR, MS, pH) at the same time, and collect real-time quantitative data under laboratory conditions of stirring, pressure and temperature. When static NMR tubes are used for studying chemical transformations, good structural and mechanistic data can be obtained, especially for reactive or labile intermediates. Care must be taken when relying on kinetic information obtained from systems that do not have sufficient mixing.
The combination of online NMR reaction monitoring and IR spectroscopy in the laboratory provides a better understanding of reaction processes and supports in-situ IR process monitoring in pilot and production plants.
This information has been sourced, reviewed and adapted from materials provided by Bruker BioSpin - NMR, EPR and Imaging.
For more information on this source, please visit Bruker BioSpin - NMR, EPR and Imaging.
Please use one of the following formats to cite this article in your essay, paper or report:
Bruker BioSpin - NMR, EPR and Imaging. (2023, April 10). Improving Reaction Understanding with Online NMR Reaction Monitoring in Chemical Route Development Applications. AZoM. Retrieved on January 30, 2024 from https://www.azom.com/article.aspx?ArticleID=12557.
Bruker BioSpin - NMR, EPR and Imaging. "Improving Reaction Understanding with Online NMR Reaction Monitoring in Chemical Route Development Applications". AZoM. 30 January 2024. <https://www.azom.com/article.aspx?ArticleID=12557>.
Bruker BioSpin - NMR, EPR and Imaging. "Improving Reaction Understanding with Online NMR Reaction Monitoring in Chemical Route Development Applications". AZoM. https://www.azom.com/article.aspx?ArticleID=12557. (accessed January 30, 2024).
Bruker BioSpin - NMR, EPR and Imaging. 2023. Improving Reaction Understanding with Online NMR Reaction Monitoring in Chemical Route Development Applications. AZoM, viewed 30 January 2024, https://www.azom.com/article.aspx?ArticleID=12557.
Do you have a question you'd like to ask regarding this article?
AZoM.com - An AZoNetwork Site
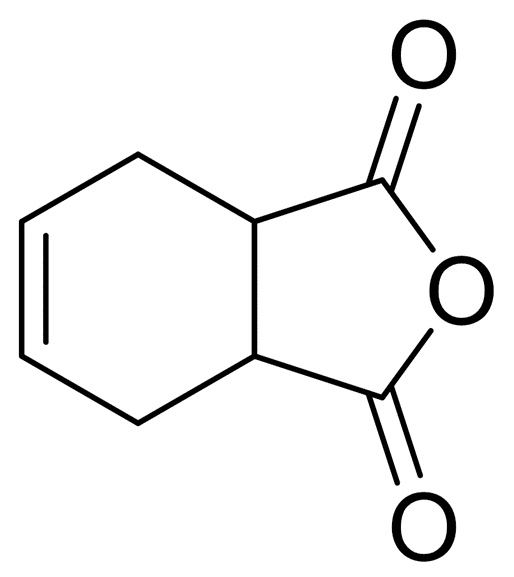
Ethylene Dimethacrylate Owned and operated by AZoNetwork, © 2000-2024