Thank you for visiting nature.com. You are using a browser version with limited support for CSS. To obtain the best experience, we recommend you use a more up to date browser (or turn off compatibility mode in Internet Explorer). In the meantime, to ensure continued support, we are displaying the site without styles and JavaScript.
Scientific Reports volume 13, Article number: 18214 (2023 ) Cite this article 1/1 Poplin 140*120/60*60
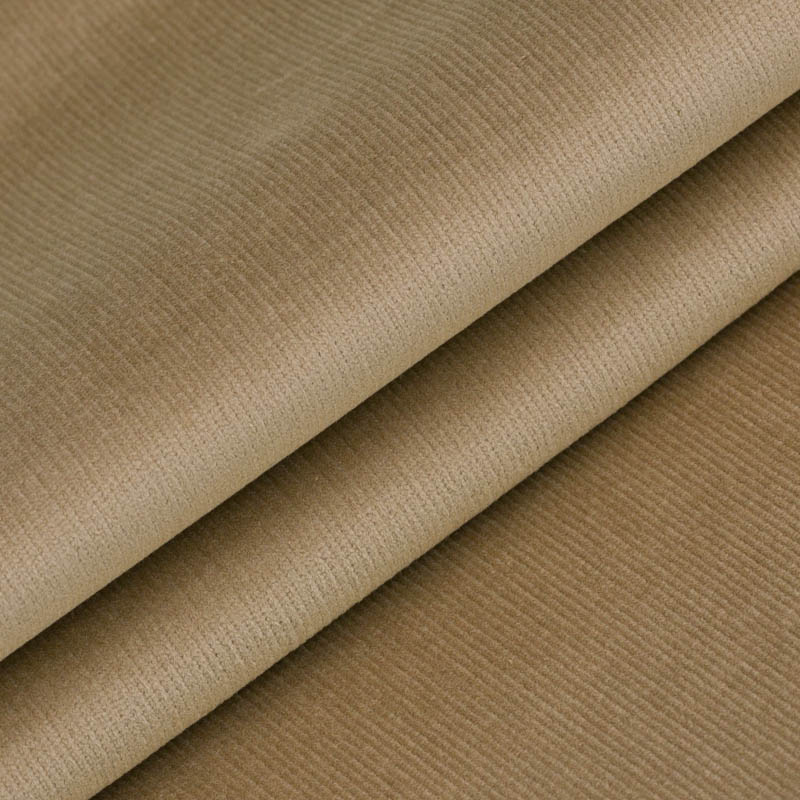
The most important uses of old fabrics include clothing, mummification, and bookbinding. However, because they are predominantly constructed of natural materials, they are particularly susceptible to physical and chemical deterioration brought on by fungi. The treatments that are typically used to preserve old textiles focus on the use of synthetic fungicides, which have the potential to be dangerous for both human health and the environment. Essential oils (EOs), which are safe for the environment and have no negative effects on human health, have been widely advocated as an alternative to conventional antifungals. Four natural fabrics—linen, cotton, wool, and silk—were utilized in the current work. The extracted EO from leaves of river red gum (Eucalyptus camaldulensis Dehnh.) were prepared at 125, 250, and 500 µL/L. Aspergillus flavus, Fusarium culmorum and Aspergillus niger were inoculated separately into the treated four fabrics with the EO at concentrations of 125, 250, and 500 µL/L or the main compounds (spathulenol and eucalyptol) at the concentrations of 6, 12, 25, and 50 µL/L and were then compared to the un-treated samples. GC–MS was used to analyze the EO chemical composition, while visual observations and scanning electron microscopic (SEM) were used to study the fungal growth inhibition. Spathulenol (26.56%), eucalyptol (14.91%), and p-cymene (12.40%) were the principal chemical components found in E. camaldulensis EO by GC–MS. Spathulenol molecule displayed the highest electrostatic potential (ESP) compared with the other primary compound, as calculated by quantum mechanics. In the untreated textile samples, SEM analysis revealed substantial proliferation of hyphae from A. flavus, F. culmorum, and A. niger. The fungal growth was completely inhibited at a concentration of 500 µL/L from the EO. Both eucalyptol and spathulenol completely inhibited the formation of the fungal spores at a concentration of 50 µL/L, although eucalyptol was more effective than spathulenol across the board for all four textiles. The results support E. camaldulensis EO functionalized textiles as an effective active antifungal agent.
The world was aware of the widespread use of textiles for a variety of tasks, the most significant of which was the production of clothing, mummification, and the binding of antiquarian books1,2,3,4. The most significant of which was the binding of the Description of Egypt collection books kept in various Egyptian museums and libraries5,6.
There are numerous sources for the raw materials that can be used in the textile industry. Natural fibers come from plants (cotton, linen, and bast fibers)7,8,9,10, from animal or natural protein fibers (wool, silk)9,11, and natural mineral fibers (asbestos)12.
Microfungi have the potential to deteriorate textile materials13,14. The following fungi have been found to be destructive to textile materials in the soil and the air are: Aspergillus, Penicillium, Alternaria, Cladosporium, Fusarium, Trichoderma, etc.15,16. Microorganisms break down fiber and cloth on average, resulting in a loss of bulk and mechanical strength17. Fungi, which cause the enzymatic degradation of cellulose and penetrate the secondary wall, are primarily responsible for the breakdown of cellulose and cellulosic textile substrates18,19,20.
Nowadays, there is high demand for fabrics with functional or specialized finishes generally, but antimicrobial treatments, in particular, to protect people from microorganisms21,22,23,24. Therefore, to prevent toxic emissions from chemicals and enhance antimicrobial activity, various additives, such as natural pigments as dyes, were utilized in the textile fabrics (cotton, wool, silk, and nylon) for clean and environmentally friendly technologies25. Silver nanoparticles (AgNPs)-treated textiles have been found to be an environmentally friendly preservative that boosts product durability and inhibits fungal development15. The maximum activity against Stemphylium solani was seen in flax pulp treated with Paraloid B-72 + Ag NP (1%)26. With the addition of copper silicate, the created biofunctionalization of nonwovens with polypropylene and biodegradable polylactide had outstanding antimicrobial capabilities against Escherichia coli and Staphylococcus aureus, and Candida albicans27. They also produced biodegradable nonwoven textiles27. In comparison to untreated fibers, cotton textiles grafted with polyacrylic acid that was immobilized organic phosphorus monomer demonstrated notable flame-retardant capabilities and altered the mechanical behavior of the cotton28. The polyester and viscose nonwovens were combined with triclosan-loaded microparticles to create a finished product that was very effective against S. aureus and K. pneumoniae29.
Natural substances including essential oils (EOs), phenolic compounds, and flavonoid compounds have been intensively studied for their antimicrobial activities when applied to organic materials like wood, paper, textiles, and parchment10,30,31,32. Textiles have become more environmentally and human-friendly by using bioactive materials and dyes as finishing agents33,34,35,36.An efficient natural antibacterial and antifungal finish for cotton textiles has been created using the bioactive compounds of Neem plants combined with extracted Aloe vera gel37. Treated cotton fabrics especially in medical textiles with lavender, thyme and clove EOs had significant antibacterial activity against, S. aureus and Escherichia coli38. Numerous EOs and their individual components have been successfully used to combat a variety of pathogenic bacteria through their promising anti-quorum sensing and anti-biofilm forming properties39,40,41,42,43.
The growth of fungus mycelium and spore germination was inhibited by a number of naturally occurring substances used for textile dyeing44. Mahonia napaulensis dyed textile pieces showed effective antifungal properties against Colletotrichum capsici, Leptosphaerulin trifoli, Alternaria brassicicola, and Helminthosporium solani in both methanolic and aqueous extracts45. Neem and Mexican daisy extracts were used to create an antimicrobial finish on cotton fabric. Both direct application and microencapsulation were used, and the results showed that the microencapsulated herbal extracts had excellent resistance to microorganisms even after 15 washings46. The treated 100% cotton fabrics with Aloe vera methanol extract exhibited antimicrobial activity against the S. aureus47. Incorporated lime EO microcapsules into the cotton fabric demonstrated strong antibacterial action against E. coli, Bacillus cereus, Salmonella typhimurium, and S. aureus48.
In order to create novel and useful applications in fields related to human health, agriculture, and the environment without causing the same secondary effects that chemicals may cause, it is important to better understand the chemical complexity and biological properties of EOs49,50,51,52. The diverse biological features of the EOs are conferred by their substantial chemical composition and considerable variance53,54,55,56,57. The main components of the EOs, however, are not the only agents in charge of these properties58,59,60. Textile fabric treated with Rosmarinus officinalis and Citrus sinensis EOs had promising antifungal efficacy against Aspergillus niger, A. flavus, C. albicans, Trichoderma viride and Epidermophyton floccosum61. Tea, and eucalyptus EOs were used to treat cotton fabrics, and promising antimicrobial effectiveness was seen47. Trichophyton interdigitale was fully inhibited from growing on cotton fabrics by the application of EOs from origanum, cinnamon, origanum-clove-orange, and clove-lavender-cinnamon62.
The primary objectives of the current work were to first use GC–MS to identify the chemical components of the EO extracted from Eucalyptus camaldulensis leaves, and then to determine the quantum mechanical properties of the principal constituents of the EO. After treating four textiles, the EO and its two primary components were tested as antifungal agents against various fungal isolates that were identified from contaminated old textiles.
This study has complied with relevant institutional, national, and international guidelines and legislation. This study does not contain any studies with human participants or animals performed by any of the authors. Four natural fabrics (linen, cotton, wool, and silk) were bought from Manstex Textile Company, Alexandria, Egypt; Misr Spinning & Weaving Company, Mahala El Kobra, Egypt; Goldentex Wool Textile Company, 10th of Ramadan, Egypt; and Awlad Khattab Company for Handmade Silk Textile, Akhmim, Egypt, respectively (Table 1).
The essential oil (EO) from leaves of Eucalyptus camaldulensis Dehnh. (Family Myrtaceae) were extracted by the hydrodistillation method using the Clevenger apparatus at the Faculty of Agriculture (EL-Shatby), Alexandria University, Alexandria, Egypt, where approximately 150 g of small pieces of leaves were put in a 2 L flask containing 1500 mL of distilled water and then connected to a Clevenger unit and heated for 3 h under refluxing50,63. The EO was collected and dried over anhydrous sodium sulfate and the EO amount was calculated based on sample weight10 as 2.44 mL/100 fresh leaves of E. camaldulensis. The collected EO was preserved at 4 °C in a refrigerator while being kept dry in an Eppendorf tube.
The EO from E. camaldulensis were analyzed for their chemical composition using Focus GC-DSQ Mass Spectrometer (Thermo Scientific, Austin, TX, USA) with a direct capillary column TR–5MS (30 m × 0.25 mm internal diameter and 0.25 µm film thickness) apparatus at Atomic and Molecular Physics Unit, Experimental Nuclear Physics Department, Nuclear Research Centre, Egyptian Atomic Energy Authority, Inshas, Cairo, Egypt. The column oven temperature was initially held at 60 °C and then increased by 5 °C/min to 250 °C with holding 2 min and then increased to 300 °C (25 °C/min). Temperature of the injector were kept at 270 °C. Helium, the carrier gas, was kept in constant flow rate of 1 mL/min. The solvent delay was 4 min, and diluted samples of 1 µL were injected automatically using Auto-sampler AS3000 coupled with GC in the split mode. EI mass spectra were collected at 70 eV ionization voltages over the range of m/z 50–550 in full-scan mode. The ion source and transfer line temperatures were set at 200 and 250 °C, respectively. By comparing the components' retention times and mass spectra to those from the WILEY 09 and NIST 11 mass spectral databases, the components were identified.
A powerful method for analyzing EO or derivatized nonvolatile molecules at lower concentrations and providing a great depth of information is coupling chromatography to MS via gas chromatography-mass spectrometry (GC–MS). This method has higher sensitivity, selectivity, and structural determination capabilities than other structural characterization methods like IR and Raman spectroscopy64.
With the help of the molecular modeling program Hyperchem7.5 (W. Thiel 2003, HyperChemTM, Release 7.5 Pro 2002), the geometry of the compounds under study has been optimized on the basis of semi-empirical calculations65. Semi-empirical calculations were carried out using the routine Modified Neglect of Diatomic Overlap (MNDO) and the Polak–Ribiere conjugated gradient algorithm66,67. For the optimized structure at the ground state and reported the molecular electrostatic potential of the active functional groups of the studied components.
The antifungal bioassays of the EO and the main two compounds, eucalyptol and spathulenol, purchased from Sigma-Aldrich (Merck), were conducted using three molds (Aspergillus flavus AFl375, Fusarium culmorum Fcu761, and Aspergillus niger Ani245) and accession numbers in Gen Bank, MH355958, MH355955, and MH355957, respectively68,69. These fungal strains were previously isolated from contaminated ancient textiles (Fig. 1A–D)70. The extracted EO from E. camaldulensis leaves was prepared at the concentrations of 125, 250, and 500 µL/L, while eucalyptol and spathulenol were prepared at 6, 12, 25, and 50 µL/L. Tween 40 (0.5 mL) was added after the EO had been diluted in 10% DMSO, in order to disseminate the EO more widely and ensure homogeneous evaporation.
Contaminated ancient textiles. (A) Coptic textile No. 1385, a wool and linen item from the third or fourth century AD (26 × 24 cm) that was placed at the Faculty of Arts Museum, Alexandria University, (B) Woolen weaved No. 1381 from the Museum of the Faculty of Arts, Alexandria University, which dates to the sixth century AD, (C) Islamic woven inscription No. 1244, written in Kufic script, placed at Museum of the Faculty of Arts, Alexandria University (Photos were taken by coauthor Ibrahim H. M. Ibrahim), and (D) The antique textile from 1822 AD that was used to tie the Description of Egypt, which is housed in Cairo, Egypt's Central Library of Manuscripts (Photo was taken by coauthor Ayman S. Taha).
Samples of linen, cotton, wool, and silk in the dimensions of 2 × 2 cm were exposed to the prepared EO and eucalyptol and spathulenol using the vapor method71,72. The treated and un-treated textiles were exposed to the fungal infestation using the three molds. The samples were inoculated with a 9-mm disc diameter from each fungus' 15-day-old PDA culture in Petri dishes and incubated for 14 days at 25 ± 1°C. Following inoculation, the growth on disc (mm) and inhibition zone (mm) for each fungus were measured 7 and 14 days after inoculation for textiles treated with EO and after 14 days for textiles treated with eucalyptol and spathulenol70,73.
The eucalyptus oil's minimal inhibitory concentrations (MICs) were examined at concentrations between 7.5 and 500 µL/L, while those for the components eucalyptol and spathulenol were studied from 1 to 50 µL/L50,74.
Fungal infestations over the four the samples textile were examined using a Scanning Electron Microscope. The samples were coated with gold in a fine coat and examined via SEM-JEOL (JFC-1100E Ion sputtering device, model JSM- 5300, JEOL Co., Tokyo, Japan) at 8 Kv26.
The effects of the EO concentrations or the main two compounds and the treated textiles with the EO as well as their interactions on the inhibition zone values and the growth of the tested fungi over the textile samples were statistically analyzed in a two-way ANOVA analysis of variance (ANOVA) using SAS software (SAS Institute, Release 8.02, Cary, North Carolina State University, Raleigh, NC, USA), and the means were compared against the control treatment. Additionally, ANOVA with three factors was used to study the significant effects of monoterpenes (eucalyptol and spathulenol) with their concentrations when applied to the four textiles on the IZ and the growth on textile samples after 14 days incubation with A. flavus, F. culmorum, and A. niger.
Figure S1 displays the identification of the investigated fibers. Longitudinal views of fibers are more distinctive and special, where linen thread has thick nodes with crossings and the typical polygonal structure of the fibers (Fig. S1a,b), whereas wool fiber has scales that are, for example, plainly visible even when covered by a thin coating of dirt (Fig. S1c)75,76,77. The cotton fibers seem like a twisted ribbon (Fig. S1d,e), compared to silk fibers longitudinal views are straight (Fig. S1f)78,79,80.
Figure S2 depicts the determined chemical composition of the EO from E. camaldulensis, and Table 2 lists 26 components. The most abundant compounds were spathulenol (26.56%), eucalyptol (14.91%), p-cymene (12.40%), crypton (7.72%), carvacrol (4.21%), phellandral (4.55%), alloaromadendrene oxide-(2) (3.22%), 4-terpineol (3.00%), cuminaldehyde (2.97%), ylangenal (2.89%), aromandendrene (2.79%), p-cymen-7-ol (1.73%), 4(15),5,10(14)-germacratrien-1-ol (1.49%), and ledol (1.22%).
The molecular mass, chemical formula, chemical structure, or quantity of the analyte can be determined using mass spectrometric analysis of the organic components found in the EO made from E. camaldulensis leaves (Table 2). The formula and chemical structure can be ascertained manually81 and/or by comparison with a reference database of spectra based on the measured m/z and their peak intensities.
In the high-throughput examination of chemicals synthesized in combinatorial libraries, such as those created to find new medicines, mass spectra (MS) are also a useful technique. Both structural data and information regarding a molecule's binding affinity can be collected if the mass spectrometer is coupled to an LC system with the right columns82. By listing the molecular and fragment ions of a chemical, it was demonstrated that the mass spectrum is actually nothing more than a depiction of the substance's molecular structure. Any molecule's structure determines its pharmacological and biological activity, and a change in structure might result in a change in activity83,84.
The principle EO components rom E. camaldulensis had their 70 eV MS recorded and explained, as illustrated in Fig. 2a–f. The spathulenol component is represented by the peak at retention time (RT) of 18.77 min (Fig. 2a), which suggests that its chemical formula is C15H24O (Table 2). The molecular ion peak was detected at m/z 220 with a relative intensity (RIn) of 10%, while and the base peak is represented by the peak at 91 (RIn = 100%). Significant fragment ions were also found at positions 205(85%), 159(80%), 147(59%), 119(85%), and 79(60%).
The 70 eV mass spectrum of (a) spathulenol component at RT 18.77 min, (b) Eucalyptol component at RT 5.44 min, (c) p-cymene component at RT 5.27 min, (d) Crypton component at RT 9.07 min, (e) carvacrol component at RT 12.07 min, and (f) Phellandral component at RT 11.39 min.
The peak at RT 5.44 min (Fig. 2b) reveals the eucalyptol component, which is indicated by the MS and has the chemical formula C10H18O. A persistent molecular ion peak was found at m/z 154 with RIn = 42%, and the base peak is found at m/z 81 with RIn = 100%. The following notable fragment ions were also found: 139 (58%), 108 (63%), and 81 (85%). The p-cymene component is seen in the MS of the peak at RT 5.27 min (Fig. 2c), which proves its chemical formula is C10H14 (Table 2). The base peak, which is represented by the peak at 119 (RIn = 100%), is the molecular ion peak, which was seen at m/z 134 with RIn = 38% (stable molecular ion). The detected fragment ion of 91 (25%) was also noteworthy.
The crypton component is represented by the MS of the peak at RT 9.07 min (Fig. 2d), indicating that its chemical formula is C9H14O (Table 2). A persistent molecular ion peak was seen at m/z 138 with RIn = 20%, while the base peak was seen at m/z 95 with RIn = 100%. Fragment ions at 96 (95%), 81 (25%), and 67 (42%), where the other notable ones that were found. The carvacrol component is represented by the peak at RT 12.07 min (Fig. 2e), which suggests that its chemical formula is C10H14O (Table 2). The base peak is represented by the peak at 135 (RIn = 100%), while the molecular ion peak was found at m/z 150 with RIn = 41% (stable molecular ion). Other major fragment ions of 107 (19%), and 91 (20%) were observed.
The phellandral component is represented by the peak at RT 11.39 min (Fig. 2f), which suggests that it has the chemical formula C10H16O (Table 2). The molecular ion peak was detected at m/z 152 with RIn = 10%, while the base peak is represented by the peak at 109 (RIn = 100%). There were also notable fragment ions of 95(38%), 81(42%), and 67(38%).
Figure 3a–f displays the MEP plots for the primary chemicals. With the exception of the p-cymene molecule (Fig. 3b), it is possible to see that the investigated molecules' regions surrounding the oxygen atoms linked to the heterocyclic ring are the most electrophilic site (red regions), as a result of the concentrated electron density. Additionally, spathulenol (Fig. 3a) has the highest electrostatic potential (ESP) compared to the other key E. camaldulensis EO compounds, which explains why it makes up the majority of this molecule in the chemical composition in the EO extracted from E. camaldulensis leaves. On the other hand, the nucleophilic site of a p-cymene molecule is represented by the areas close to the carbon atom (green regions).
The optimized structures and electrostatic potential 3D mapped using the HyperChem program using MNDO method for the studied components. (a) Spathulenol, (b) p-Cymene, (c) Eucalyptol, (d) Crypton, (e) Carvacrol; and (f) Phellandral.
Semi-empirical calculations were carried out using the routine MNDO and Polak–Ribiere conjugated gradient algorithm and the program MNDO were used to do semi-empirical computations. The molecular charge distribution is unaffected by the external test charge, making molecular electrostatic potential (MEP) a useful tool for determining the reactive sites toward positively or negatively charged reactants and enabling the establishment of the hydrogen bonding and structure–activity relationships of the molecule85. Quantum chemical calculations have shown that the electrostatic potential, electronegativity, partial charges, and dipole moment are strongly correlated86. By plotting the total density surface on the electrostatic potential energy surface and showing the size, shape, charge density, and reactive sites of the molecules, MEP offers a visual way to comprehend the relative polarity of a molecule.
After 7, and 14 days of incubation, Table S1 displays the significant impacts of EO concentrations, the types of textiles treated with the EO, and their interactions on the inhibition zones (IZs) and the growth of Aspergillus flavus, Fusarium culmorum, and A. niger. As can be seen, the ANOVA test revealed significant differences between the level concentrations of E. camaldulensis EO, the types of textiles treated with the EO, and their interactions, with the exception of the interaction between EO concentrations and treatments on the growth of A. flavus on samples after 14 days of incubation (p = 0.5093) and the impact of textiles treated with the EO on the IZs after 7 days of incubation against F. culmorum (p = 0.0879).
Table 3 and Fig. S3 show the IZs (mm) and the growth on the textile samples treated with EO after 7 and 14 days of A. flavus incubation. The treated Silk, Linen, and Cotton fabrics showed the highest IZs at 500 μL/L after 7 days of incubation, with values of 8.33, 5.66, and 4 mm, respectively. These values fell to 7, 4.33, and 3.33 mm, respectively, after 14 days of incubation. While wool materials had a 0.33 mm growth after 14 days of A. flavus incubation, treated cotton, linen, and silk with 500 µL/L of E. camaldulensis EO did not exhibit any visible growths. After 14 days of incubation and in the control treatments, the growth over the textiles made of cotton, linen, silk and wool was 20, 19.66, 19.66, and 18 mm, respectively.
After 7 and 14 days of incubation, Table 4 and Fig. S4 demonstrate the E. camaldulensis EO’s bioactivity against the development of F. culmorum. The maximum IZ values of 6, 4.33, 3.66, and 3.33 mm against the growth of F. culmorum were seen after 7 days of incubation, when silk, linen, cotton and wool samples, respectively, were treated with 500 µL/L of EO concentration. However, after 14 days of incubation, these values fell to 5, 4, 3.33, and 2.66 mm, respectively.
After 7 days from inoculation, F. culmorum fungal growth ranged from 19 to 19.66 mm in cotton, linen, silk, and wool in the control treatment, and from 19.66 to 19.33 mm in samples treated with 125 µL/L of E. camaldulensis EO. These values roughly reached 20 mm after 14 days of incubation. After 7 or 14 days of incubation, no fungus was discovered over the textile samples that had been treated with 500 µL/L of E. camaldulensis EO.
The antifungal activity of the textiles treated with E. camaldulens EO against the growth of A. niger is shown in Table 5 and Fig. S5.The treated cotton, linen, silk and wool with 500 µL/L of E. camaldulensis EO revealed IZ values of 8.66, 6.33, 6, and 5.33 mm, respectively, after 7 days of incubation with A. niger. After 14 weeks of incubation, these values marginally dropped to 6.66, 4.66, 5.33, and 3.66 mm, respectively. After 14 days of incubation, the fungal growth in untreated cotton, linen, silk, and wool reached 19.66, 20, 20, and 19.33 mm, respectively. This growth was halted in textiles treated with 500 µL/L of E. camaldulensis EO.
The SEM was used to compare the patterns of fungal development in the treated and untreated textiles by E. camaldulensis EO inside and around the fibers. A. flavus showed extensive growth in cotton samples that had not been treated with EO (Fig. 4a); this growth nay have deceased in cotton samples that had been treated with 250 µL/L of E. camaldulensis EO (Fig. 4b); however the mycelial net of A. flavus was still visible in cotton samples that had been treated with 125 µL/L of E. camaldulensis EO (Fig. 4c).
SEM images of cotton samples inoculated with: A. flavus without treatment (a), A. flavus with 250 µL/L of E. camaldulensis EO (b), A. flavus with 125 µL/L of E. camaldulensis EO (c), F. culmorum without treatment (d), F. culmorum with 250 µL/L of E. camaldulensis EO (e), A. flavus with 125 µL/L of E. camaldulensis EO, (f), A. niger without treatment (g), A. niger with 250 µL/L of E. camaldulensis EO (h), and with 125µL/L of E. camaldulensis EO (i). The growth of fungal mycelia dependent on EO treatment concentrations is indicated by solid arrows, while the longitudinal twists of cotton fiber are indicated by dotted arrows.
The same trend was observed under SEM examination, where a huge growth of F. culmorum (Fig. 4d) and A. niger (Fig. 4g) was found in untreated cotton samples and these growths were significantly decreased as the samples treated with 250 µL/L of E. camaldulensis EO (Fig. 4e,h) with some growths were shown in samples treated with 125 µL/L E. camaldulensis EO (Fig. 4f,i).
Without any treatments, fungal mycelial grew rapidly on linen samples infected with A. flavus (Fig. 5a), F. culmorum (Fig. 5d), and A. niger (Fig. 5g). When the linen samples were treated with 250 µL/L of E. camaldulensis EO (Fig. 5b,e,h), the fungal growth was obviously suppressed, whereas some growth was prevented when the linen samples were treated with 125 µL of E. camaldulensis EO (Fig. 5c,f,i).
SEM images of linen samples inoculated with A. flavus without treatment (a), A. flavus with 250 µL/L of E. camaldulensis EO (b), A. flavus with 125 µL/L of E. camaldulensis EO (c), F. culmorum without treatment (d) F. culmorum with 250 µL/L of E. camaldulensis EO (e), F. culmorum with 125 µL/L of E. camaldulensis EO (f), A. niger without treatment (g), A. niger 250 µL/L of E. camaldulensis EO (h), and A. niger with 125 µL/L of E. camaldulensis EO (i). Solid arrows point to the development of fungus mycelia in response to EO concentrations, whereas dot-filled arrows depict the distinctive nodes of flax fibers in a longitudinal view.
A. flavus growth on untreated wool samples can be seen under a microscope (Fig. 6a), and growth on wool samples treated with 250 µL/L of E. camaldulensis EO dramatically decreased with destruction in the mycelial structures (Fig. 6b). The amount of fungal development that was reduced in the treated wool with 125 µL/L of E. camaldulensis EO was not noticeably greater (Fig. 6c).
SEM images of wool samples inoculated with A. flavus without treatment (a), A. flavus with 250 µL/L of E. camaldulensis EO (b), A. flavus with 125 µL/L of E. camaldulensis EO (c), F. culmorum without treatment (d); F. culmorum with 250 µL/L of E. camaldulensis EO (e), F. culmorum with 125 µL/L of E. camaldulensis EO (f), A. niger without treatment (g), A. niger 250 µL/L of E. camaldulensis EO (h), and A. niger with 125 µL/L of E. camaldulensis EO (i). The growth of fungus-based mycelia is indicated by solid arrows, while the distinct nods of wool fiber's longitudinal perspective are indicated by dotted arrows.
Similar to how F. culmorum colonies were seen on wool samples without treatment (Fig. 6d), they significantly decreased after being treated with 250 µL/L of E. camaldulensis EO (Fig. 6e), and F. culmorum hyphae were visible on wool samples treated with 125 µL/L of E. camaldulensis EO (Fig. 6f). The heavy and intensive growth of A. niger on the untreated wool samples is clearly visible in Fig. 6g, while the wool treated with 250 µL/L of E. camaldulensis EO (Fig. 6h) or 125 µL/L of E. camaldulensis EO (Fig. 6i) showed a reduction in conidia.
Figure 7 compares the silk textile that had been previously treated with E. camaldulensis EO and had been inoculated with A. flavus, F. culmorum, and A. niger to the untreated material. The untreated silk samples showed massive expansion of the mycelial net of A. flavus (Fig. 7a), F. culmorum (Fig. 7d), and A. niger (Fig. 7g). As the silk samples treated with 250 µL/L of E. camaldulensis EO against A. flavus (Fig. 7b), F. culmorum (Fig. 7e) and A. niger (Fig. 7h), significant decreases in this net of fungal hyphae were seen. Silk samples treated with 125 µL/L of E. camaldulensis EO and inoculated with A. flavus, F. culmorum, and A. niger (Fig. 7c, h, and i, respectively) revealed some reduction in the fungal growth as well.
SEM images of silk samples inoculated with A. flavus without treatment (a), A. flavus with 250 µL/L of E. camaldulensis EO (b), A. flavus with 125 µL/L of E. camaldulensis EO (c), F. culmorum without treatment (d); F. culmorum with 250 µL/L of E. camaldulensis EO (e), F. culmorum with 125 µL/L of E. camaldulensis EO (f), A. niger without treatment (g), A. niger 250 µL/L of E. camaldulensis EO (h), and A. niger with 125 µL/L of E. camaldulensis EO (i). The growth of fungus mycelia based on EO concentration treatments is indicated by solid arrows; the longitudinal view of silk fibers is indicated by dotted arrows.
Table S2 demonstrate the significant effects of monoterpenes’ concentrations, textile types, monoterpenes, monoterpenes × concentrations, monoterpenes × textile types, concentrations × textile types and monoterpenes × concentrations × textile types on the IZ and the growth on textile samples after 14 days incubation with A. flavus, F. culmorum, and A. niger. Table 6 and Fig. 8 demonstrate the antifungal efficacy of eucalyptol and spathulenol against the development of A. flavus. When applied to wool fabric, eucalyptol at 50 µL/L revealed the maximum inhibition zone (6.33 mm), followed by eucalyptol at 50 µL/L (6 mm) when applied to cotton fabric. Eucalyptol at 50 µL/L applied to linen observed IZ (5.33 mm), spathulenol at 50 µL/L applied to wool revealed IZ of 5.333 mm, and spathulenol at 50 µL/L applied to cotton exhibited IZ value of 5 mm. After 14 days of incubation, eucalyptol and spathulenol at 50 µL/L and 25 µL/L revealed no development in the textile samples.
Visual observation of the effects of eucalyptol and spathulenol when treated textiles against the growth of A. flavus. (Photos were taken by coauthor Wael A. A. Abo-Elgat). S: Silk; W: Wool; L: Linen; and C: Cotton.
Table 6 and Fig. 9 depict the antifungal action of eucalyptol and spathulenol against the development of F. culmorum. After 14 days, the four fabrics that had been exposed to both chemicals exhibited no signs of F. culmorum growth. The four fabrics treated with eucalyptol and spathulenol at 50, 25, and 12 µL/L exhibited no signs of microbial development. After 14 days of incubation, eucalyptol (applied at 50 µL/L to linen, cotton, wool, and silk) and spathulenol (applied at 50 µL/L to silk fabric) both demonstrated the highest IZ value (5.33 mm) against F. culmorum with no growth on them. Additionally, spathulenol revealed an IZ value of 5 mm against F. culmorum growth when applied to cotton and wool fibers at a rate of 50 µL/L.
Visual observation of the effects of eucalyptol and spathulenol when treated textiles against the growth of F. culmorum. (Photos were taken by coauthor Wael A. A. Abo-Elgat). S: Silk; W: Wool; L: Linen; and C: Cotton.
Table 6 and Fig. 10 show the antifungal action of eucalyptol and spathulenol against the growth of A. niger when applied to four fabrics. Eucalyptol applied to cotton, wool, and silk at 50 µL/L demonstrated weak efficacy against A. niger growth, with minor IZ values (0.33 mm) mm detected. When applied to the four textiles where A. niger growth was at its strongest, other concentrations of eucalyptol and spathulenol showed little effect.
Visual observation of the effects of eucalyptol and spathulenol when treated textiles against the growth of A. niger. (Photos were taken by coauthor Wael A. A. Abo-Elgat). S: Silk; W: Wool; L: Linen; and C: Cotton.
The minimum inhibitory concentrations (MICs) of the EO were observed to be 250, 500, and 250 µL/L, and 12, 50, and 2 µL/L when using the eucalyptol compound, while it was 25, > 50, and 2 µL/L when using spathulenol, against the growth of growth of A. flavus, F. culmorum, and A. niger, respectively.
The EO from E. camaldulensis and its bioactivity against the growth of three molds when applied to and functionalized on four textiles (linen, cotton, wool, and silk), as well as the main two compounds, eucalyptol and spathulenol, have been established and investigated based on the results presented above. When the EO evaluated by the GC–MS, the presence of primary bioactive chemicals were spathulenol, eucalyptol, p-cymene, crypton, carvacrol, phellandral, alloaromadendrene oxide-(2), 4-terpineol, cuminaldehyde, ylangenal, aromandendrene, p-cymen-7-ol, 4(15),5,10(14)-germacratrien-1-ol, and ledol.
All of the fabric samples indicated an increased quantity of fungi after just seven days. Other authors have already provided evidence of the potential intense development of fungi and bacteria on natural fiber fabrics, leading to their biodegradation87,88,89,90. The hyphae of fungi were seen under the SEM inspection in the untreated fabrics with EO and had a large amount of growth.. In the study by Crawford et al.91, SEM was used to observe the fracture planes and failure modes of the biocomposite samples as well as to see the hyphal growth of Chaetomium globosum on the natural fibers (non-woven flax, hemp, and oriented flax or hemp fiber (50%) and polypropylene fiber [50%]). Natural fibers can become deteriorated by microorganisms as a result of the production of hydrolytic extracellular enzymes such cellulase and lignase92,93,94. After a 35-day period of microbial incubation, flax fiber was definitely destroyed more than cotton fiber by the fungi Trichoderma viride, Aspergillu niger, and Penicillium funiculosum95. The majority of the fungi were recovered from the flax of the linen objects, which was further supported by their significant enzyme activity, particularly beta-glucosidase96. Selected isolates from historical textiles, including Penicillium, Aspergillus, and Cladosporium, also exhibited amylase activity, which is relevant since starch can be used to fill or glue textile fabrics96.
The growth of the molds A. niger, T. viride, and Penicillium funiculosum was found to be well-suited to cellulose fibers, and the bacterial growth of Bacillus megaterium, Pseudomonas fluorescens, and Streptomyces sp. to silk fabrics95. Compared to proteinaceous fibers, cellulose fibers are more sensitive to microbial breakdown. As a result, it's essential to maintain the necessary environmental conditions in museums because they cannot stop the growth of xerophilic fungi96. Since common airborne fungus species may pose a threat to textiles that have been preserved, using EOs and their primary constituents in vapor form may be an effective tactic to inhibit or prevent fungal growth. One great source for this is the EO from E. camaldulensis and its primary constituents.
The main compounds from E. camaldulensis EO were 1,8-cineole (eucalyptol), α-pinene, α-phellandrene, and p-cymene, which produced a complete inhibition to the mycelial growth of five Fusarium spp. at a concentration of 7–8 μL/mL after five days of incubation97. In another study, the use of E. camaldulensis EO prevented the growth of fungi that colonized as wood rot fungi, household molds, or plant pathogenic fungi including C. globosum, Fusarium oxysporum, A. niger, Thanatephorus cucumeris, and Rhizopus oryzae98. At a concentration of 5 mg/mL of E. camaldulensis EO, the mycelial growth of F. oxysporum was suppressed to 84% of its maximum potential98. With neither p-cymene or cryptone discovered in the plant collected from Taiwan, the leaf EO of E. camaldulensis revealed the existence of 1,8-cineole as a dominating compound and demonstrated fungal growth inhibition against A. clavatus and A. niger at 79.66% and 24.05%, respectively, after 7 days of inoculation99. At 20 μL/plate, the EOs from eleven E. camaldulensis from Sardinia, Italy, totally inhibited A. flavus. These compounds included p-cymene, 1,8-cineole, β-phellandrene, spathulenol and cryptone100. The primary compounds found in E. camaldulensis EO with significant antifungal activity against F. culmorum and F. solani were p-cymene, γ-eudesmol, L-linalool, and piperitone101.
The 1,8-cineole was found as the primary compound in the EOs of E. largiflorens, E. microtheca, E. oleosa, E. spathulata and E. torquata, and they showed substantial antifungal activity against Aspergillus flavus, A. parasiticus, A. niger, Penicillium chryzogenum, and P. citrinum102. When applied to some wood samples, E. camaldulensis leaf EO, which primarily contains eucalyptol, α-pinene, and γ-terpinene demonstrated powerful antifungal activity against C. globosum no inhibition against A. niger, and Trichoderma viride and only minimal inhibition of Fusarium subglutinans at high concentration72.
The amount of 1,8-cineole, p-cymene, α-pinene, and cryptone and the antibacterial activity were found to be somewhat correlated103. The increase p-cymene concentration in leaf EO may be the cause of its antimicrobial activity104. According to studies by Bowers and Locke105, Inouye et al.106, and Angelini et al.107, Melaleuca alternifolia EO has an inhibitory effect on phytopathogenic fungi like A. fumigatus, F. solani, P. expansum, Botrytis cinerea, R. oryzae, Blumeria graminis, and Pyrenophora graminea. Two mycotoxigenic fungi, F. graminearuma, and F. culmorum, were subjected to the effects of EO and its main purified components (terpinen-4-ol, terpinen, and 1,8-cineole)108.
The biocidal (antibacterial, antiviral, antifungal and insect repellent) uses and therapeutic benefits are possible with the EO-based bio-functional textiles109,110. Using 1%, 3%, and 5% of each EO from rosemary and orange which contain the abundant compounds (eucalyptol, camphor, and α–pinene), and (limonene, limonene oxide, α–pinene, and β-phellandrene), respectively, treated textile substrate (56% cotton/44% polyester) reduced rates of 22.12%, and 51.45% against A. niger, and 18.3%, and 60.57% against A. flavus, respectively61.
Application of EO from Thyme applied to linen-cotton blended fabric at an 8% concentration in methanol revealed possible antimicrobial activity with no mold growth and or appreciable loss of braking force111. The growth of A. niger mold could be completely inhibited by applying 2% to 25% of lemongrass EO on polyester fabric discs112. The best antimicrobial activity against Staphylococcus aureus, Klebsiella Pneumoniae and Candida albicans is also seen in treated or colored cotton, wool, silk, and nylon fabrics that have curcumin extract added25.
With an acrylate-based binder to secure the microcapsules to the fabric, rose and sage EO microcapsules were applied to 100% cotton and 50% cotton/50% polyester woven fabrics, respectively, and demonstrated improved fabric durability through washing and handling as well as good candidates for providing biological properties113. When applied to fabrics, the EOs from Patchouli114,115, Artemsia argyi116 and moxa117 exhibits eco-friendly antibacterial action. Cotton fabric treated with rosemary has shown effective antibacterial action against Staphylococcus aureus and Escherichia coli118. Cinnamomum zeylanicum EO-functionalized heritage textiles using vapor phase showed good antimicrobial activities against A. niger, Penicillium funiculosum, Trichoderma viride, Streptomyces rutgersensis, Bacillus megaterium, and Pseudomonas fluorescens119.
The activity of the EO could be related to the main compounds eucalyptol and spathulenol. Eucalyptol was observed toxic effects on in vitro mycelium growth of F. subglutinans, F. cerealis, F. verticillioides, F. proliferatum, F. oxysporum, F. sporotrichioides, A. tubingensis, A. carbonarius, Alternaria alternata and Penicillium sp.120. Eucalyptol reduced the growth of plant pathogenic fungus Botrytis fabae significantly121. Eucalyptol was shown to have comparable fungicide properties against F. oxysporum f. sp. albedinis122. Spathulenol was observed potential activity against several yeasts and filamentous fungi like Tricophyton mentagrophytes and Microsporum gypseum123. The EO of Psidium guineense and spathulenol were observed potential antioxidant, anti-inflammatory, antiproliferative and antimycobacterial activities124.
It is significant to remember that in vivo toxicity is not necessarily equivalent to in vitro bioactivity data. This work thus paves the way for future investigations into the effects of medicines over a lengthy period of time, or what is known as shelf life. study of the speeds at which oil vapors employed in treatments evaporate and are absorbed by textile fibers. Future research is advised to measure the characteristics of textile fibers and the degree to which treatments alter them, as well as the application of treatments to numerous other types of textile fibers. Studying mixes of oils in various proportions and concentrations, as well as researching various application techniques, can help us better understand various types and sources of oils as well as their biological resistance capabilities.
One of the future visions of the objectives of the study is the extent to which natural extracted oils can be applied in protecting ancient textiles preserved in museums, libraries, and inside display cases. Using fumigation of the extracted materials inside the displays to eliminate biological growths. While providing future protection for the textiles from the effects of fungal damage.
The EO from E. camaldulensis leaves and its two primary components, eucalyptol and spathulenol, were used to bio-functionalize four fabrics (linen, cotton, wool, and silk). Spathulenol molecule has the highest electrostatic potential, followed by eucalyptol, according to quantum chemistry calculations. The EO and the two major chemicals were specifically tested for their capacity to inhibit the growth of the three typical fungal contaminants of textiles, Aspergillus flavus, Fusarium culmorum, and Aspergillus niger. The vapor phase of E. camaldulensis EO and eucalyptol demonstrated a potent inhibitor of fungal growth. This indicates the potential for using EO or eucalyptol as natural biocides during in vitro and in situ applications to prevent the growth of fungi by substituting the most prevalent toxic biocide typically employed in the conservation of cultural material stored in libraries and museums, such as textiles. Subsequently and since all of the experiments were conducted in vitro, other variables like temperature and relative humidity on a wide scale would undoubtedly have an effect on the development and other characteristics of the textile. As a result, this study will allow for further investigation to determine the effect of essential oils as effective antimicrobials on the conserved antique textiles in museums throughout the world.
All data generated or analyzed during this study are included in this published article.
Ivana, S. & Stana, K. in Textiles for Advanced Applications (eds Kumar Bipin & Thakur Suman) Ch. 1 (IntechOpen, 2017).
Mather, R. R. & Wardman, R. H. The Chemistry of Textile Fibres (Royal Society of Chemistry, 2015).
Gameson, R. The material fabric of early British books. The Cambridge history of the book in Britain 1, 13-93 (2012).
Clarkson, C. A Hitherto Unrecorded English Romanesque Book Sewing Technique. Roger Powell, the Complete Binder: Liber Amicorum. Turnhout: Brepols, 215–239 (1996).
Thompson, J. Edward William Lane’s “Description of Egypt”. Int. J. Middle East Stud. 28, 565–583. https://doi.org/10.1017/S0020743800063832 (1996).
Lane, E. W. Description of Egypt: Notes and Views in Egypt and Nubia, Made During the Years 1825, 26, 27, and 28: Chiefly Consisting of a Series of Descriptions and Delineations of the Monuments, Scenery, &c. of Those Countries (American University in Cairo Press, 2000).
Ali, A. et al. Hydrophobic treatment of natural fibers and their composites—A review. J. Ind. Text. 47, 2153–2183. https://doi.org/10.1177/1528083716654468 (2016).
Peponi, L., Biagiotti, J., Torre, L., Kenny, J. M. & Mondragòn, I. Statistical analysis of the mechanical properties of natural fibers and their composite materials. I. Natural fibers. Polym. Compos. 29, 313–320. https://doi.org/10.1002/pc.20408 (2008).
Salem, M. Z. M., Ibrahim, I. H. M., Ali, H. M. & Helmy, H. M. Assessment of the use of natural extracted dyes and pancreatin enzyme for dyeing of four natural textiles: HPLC analysis of phytochemicals. Processes 8, 59. https://doi.org/10.3390/pr8010059 (2020).
Taha, A. S., Abo-Elgat, W. A. A., Fares, Y. G. D. & Salem, M. Z. M. Isolated essential oils as antifungal compounds for organic materials. Biomass Convers. Biorefinery https://doi.org/10.1007/s13399-022-02815-4 (2022).
Xueliang, X. Animal Fibers: Wool. Handbook of Fibrous Materials, 2 Volumes: Volume 1: Production and Characterization/Volume 2: Applications in Energy, Environmental Science and Healthcare (2020).
Di Giuseppe, D. et al. Mineral fibres and asbestos bodies in human lung tissue: A case study. Minerals 9, 618. https://doi.org/10.3390/min9100618 (2019).
Article CAS ADS Google Scholar
Rădulescu, H. C. et al. Biodeteriogenic capacity of a microfungal species isolated from textile cultural heritage items on contemporary wool materials. Proc. Rom. Acad. Ser. B 30, 167–171 (2018).
Beata, G. Historical textiles—A review of microbial deterioration analysis and disinfection methods. Textile Res. J. 87, 2388–2406. https://doi.org/10.1177/0040517516669076 (2017).
Article CAS ADS Google Scholar
Salem, M. Z. M. Chapter 9: Silver nanoparticle applications in wood, wood-based panels, and textiles. In Silver Nanomaterials for Agri-Food Applications (ed. Abd-Elsalam, K. A.) 219–234. https://doi.org/10.1016/B978-0-12-823528-7.00006-8 (Elsevier, 2021).
Tomšič, B., Klemenčič, D., Simončič, B. & Orel, B. Influence of antimicrobial finishes on the biodeterioration of cotton and cotton/polyester fabrics: Leaching versus bio-barrier formation. Polym. Degrad. Stab. 96, 1286–1296. https://doi.org/10.1016/j.polymdegradstab.2011.04.004 (2011).
Rana, S., Pichandi, S., Parveen, S. & Fangueiro, R. Chapter 4: Biodegradation Studies of Textiles and Clothing Products. In Roadmap to Sustainable Textiles and Clothing: Environmental and Social Aspects of Textiles and Clothing Supply Chain (ed. Muthu, S. S.) 83–123. https://doi.org/10.1007/978-981-287-110-7_4 (Springer, 2014).
Arshad, K., Skrifvars, M., Vivod, V., Valh, J. & Voncina, B. Biodegradation of natural textile materials in soil.Tekstilec 57, 118–132 (2014).
Gutarowska, B., Pietrzak, K., Machnowski, W. & Milczarek, J. M. Historical textiles—A review of microbial deterioration analysis and disinfection methods. Text. Res. J. 87, 2388–2406. https://doi.org/10.1177/0040517516669076 (2016).
Szostak-Kotowa, J. Biodeterioration of textiles. Int. Biodeterior. Biodegrad. 53, 165–170. https://doi.org/10.1016/S0964-8305(03)00090-8 (2004).
Shah, J. N., Padhye, R. & Pachauri, R. D. Studies on UV protection and antimicrobial functionality of textiles. J. Nat. Fibers 19, 6810–6821. https://doi.org/10.1080/15440478.2021.1932678 (2022).
Lam, Y.-L., Kan, C.-W. & Yuen, C.-W.M. Developments in functional finishing of cotton fibres—Wrinkle-resistant, flame-retardant and antimicrobial treatments. Text. Progress 44, 175–249. https://doi.org/10.1080/00405167.2012.735517 (2012).
Bhandari, V., Jose, S., Badanayak, P., Sankaran, A. & Anandan, V. Antimicrobial finishing of metals, metal oxides, and metal composites on textiles: A systematic review. Ind. Eng. Chem. Res. 61, 86–101. https://doi.org/10.1021/acs.iecr.1c04203 (2022).
Kamboj, A., Jose, S. & Singh, A. Antimicrobial activity of natural dyes—A comprehensive review. J. Nat. Fibers 19, 5380–5394. https://doi.org/10.1080/15440478.2021.1875378 (2022).
Gawish, S., Mashaly, H. M., Helmy, H. M., Ramadan, A. M. & Farouk, R. Effect of mordant on UV protection and antimicrobial activity of cotton, wool, silk and nylon fabrics dyed with some natural dyes. J. Nanomed. Nanotechnol. 8, 421. https://doi.org/10.4172/2157-7439.1000421 (2017).
Abo Elgat, W. A. et al. Evaluation of the mechanical, physical, and anti-fungal properties of flax laboratory papersheets with the nanoparticles treatment. Materials 13, 363. https://doi.org/10.3390/ma13020363 (2020).
Article CAS PubMed PubMed Central ADS Google Scholar
Sojka-Ledakowicz, J., Chruściel, J., Kudzin, MH, Łatwińska, M. & Kiwała, M. Antimicrobial functionalization of textile materials with copper silicate.Fiber Text.Eastern European5, 151–156.https://doi.org/10.5604/12303666.1215541 (2016).
Bouasria, A. et al. Advances in polymer coating for functional finishing of textiles. Front. Text. Mater. Polym. Nanomater. Enzymes Adv. Modif. Tech. https://doi.org/10.1002/9781119620396.ch3 (2020).
Karaszewska, A. et al. Preparation and properties of textile materials modified with triclosan-loaded polylactide microparticles. Polym. Adv. Technol. 28, 1185–1193. https://doi.org/10.1002/pat.4030 (2017).
Corbu, V. M. et al. Demonstration of Allium sativum extract inhibitory effect on biodeteriogenic microbial strain growth, biofilm development, and enzymatic and organic acid production. Molecules 26, 7195. https://doi.org/10.3390/molecules26237195 (2021).
Article CAS PubMed PubMed Central Google Scholar
Corbu, V. M. et al. Eco-friendly solution based on Rosmarinus officinalis hydro-alcoholic extract to prevent biodeterioration of cultural heritage objects and buildings. Int. J. Mol. Sci. 23, 11463. https://doi.org/10.3390/pr11030718 (2022).
Article CAS PubMed PubMed Central Google Scholar
Tiwari, V., Singh, R. & Pandey, A. Review of lignolytic fungi invading wood, and leather and control of selected fungi by using volatile essential oil of plant origin. Biochem. Cellular Arch. 22, 3693–3707 (2022).
Shabbir, M., Yusuf, M. & Mohammad, F. Insights into functional finishing agents for textile applications. In Handbook of Textile Coloration and Finishing (eds Shahid, M. et al.) 97–115 (Studium Press, 2017).
Jugreet, B. S., Suroowan, S., Rengasamy, R. R. K. & Mahomoodally, M. F. Chemistry, bioactivities, mode of action and industrial applications of essential oils. Trends Food Sci. Technol. 101, 89–105. https://doi.org/10.1016/j.tifs.2020.04.025 (2020).
Morais, D. S., Guedes, R. M. & Lopes, M. A. Antimicrobial approaches for textiles: From research to market. Materials 9, 498. https://doi.org/10.3390/ma9060498 (2016).
Article CAS PubMed PubMed Central ADS Google Scholar
Shahid ul, I., Shahid, M. & Mohammad, F. Perspectives for natural product based agents derived from industrial plants in textile applications—A review. J. Clean. Prod. 57, 2–18. https://doi.org/10.1016/j.jclepro.2013.06.004 (2013).
Khurshid, M. F., Ayyoob, M., Asad, M. & Shah, S. N. H. Assessment of eco-friendly natural antimicrobial textile finish extracted from Aloe vera and neem plants. Fibres Text. Eastern Eur. 6, 120–123. https://doi.org/10.5604/12303666.1172176 (2015).
El-Molla, M., El-Ghorab, A. & El-Massry, K. Preparation and characteristics of eco-friendly essential oils and its utilization for finishing cotton fabrics. Int. J. Sci. Res. 6, 4–13. https://doi.org/10.21275/ART20177649 (2015).
Camele, I., Elshafie, H. S., Caputo, L. & De Feo, V. Anti-quorum sensing and antimicrobial effect of mediterranean plant essential oils against phytopathogenic bacteria. Front. Microbiol. 10, 2619. https://doi.org/10.3389/fmicb.2019.02619 (2019).
Article PubMed PubMed Central Google Scholar
Elshafie, H. S. & Camele, I. An overview of the biological effects of some mediterranean essential oils on human health. BioMed. Res. Int. 2017, 9268468. https://doi.org/10.1155/2017/9268468 (2017).
Article CAS PubMed PubMed Central Google Scholar
Lim, A. C. et al. Chemical composition, antioxidant, antibacterial, and antibiofilm activities of Backhousia citriodora essential oil. Molecules 27, 4895. https://doi.org/10.3390/molecules27154895 (2022).
Article CAS PubMed PubMed Central Google Scholar
Ghannay, S., Aouadi, K., Kadri, A. & Snoussi, M. GC–MS profiling, Vibriocidal, Antioxidant, Antibiofilm, and Anti-Quorum sensing properties of Carum carvi L. Essential Oil: In vitro and in silico approaches. Plants 11, 1072. https://doi.org/10.3390/plants11081072 (2022).
Article CAS PubMed PubMed Central Google Scholar
D’Aquila, P., Sena, G., Crudo, M., Passarino, G. & Bellizzi, D. Effect of Essential Oils of Apiaceae, Lamiaceae, Lauraceae, Myrtaceae, and Rutaceae Family Plants on Growth, Biofilm Formation, and Quorum Sensing in Chromobacterium violaceum, Pseudomonas aeruginosa, and Enterococcus faecalis. Microorganisms 11, 1150. https://doi.org/10.3390/microorganisms11051150 (2023).
Article CAS PubMed PubMed Central Google Scholar
Gulati, R., Sharma, S. & Sharma, R. K. Antimicrobial textile: Recent developments and functional perspective. Polym. Bull. 79, 5747–5771. https://doi.org/10.1007/s00289-021-03826-3 (2022).
Bajpai, D. & Vankar, P. S. Antifungal textile dyeing with Mahonia napaulensis DC leaves extract based on its antifungal activity. Fibers Polym. 8, 487. https://doi.org/10.1007/BF02875870 (2007).
Thilagavathi, G. & Bala, S. K. Microencapsulation of herbal extracts for microbial resistance in healthcare textiles. Indian J. Fibre Text. Res. 32, 351–354 (2007).
Jothi, D. Experimental study on antimicrobial activity of cotton fabric treated with aloe gel extract from Aloe vera plant for controlling the Staphylococcus aureus (bacterium). Afr. J. Microbiol. Res. 3, 228–232 (2009).
Wijesirigunawardana, P. B. & Perera, B. G. K. Development of a cotton smart textile with medicinal properties using lime oil microcapsules. Acta Chim. Slov. 65, 150–159. https://doi.org/10.17344/acsi.2017.3727 (2018).
Article CAS PubMed Google Scholar
Mohamed, A. A. et al. Antibacterial activity of three essential oils and some monoterpenes against Ralstonia solanacearum phylotype II isolated from potato. Microb. Pathog. 135, 103604. https://doi.org/10.1016/j.micpath.2019.103604 (2019).
Article CAS PubMed Google Scholar
Shakam, H. M., Mohamed, A. A. & Salem, M. Z. M. Down-regulatory effect of essential oils on fungal growth and Tri4 gene expression for some Fusarium oxysporum strains: GC–MS analysis of essential oils. Arch. Phytopathol. Plant Prot. 55, 951–972. https://doi.org/10.1080/03235408.2022.2064081 (2022).
Abd-Elkader, D. Y. et al. Post-harvest enhancing and Botrytis cinerea Control of strawberry fruits using low cost and eco-friendly natural oils. Agronomy 11, 1246. https://doi.org/10.3390/agronomy11061246 (2021).
Camele, I., Gruľová, D. & Elshafie, H. S. Chemical composition and antimicrobial properties of Mentha× piperita cv‘Kristinka’essential oil. Plants 10, 1567. https://doi.org/10.3390/plants10081567 (2021).
Article CAS PubMed PubMed Central Google Scholar
Valerio, F. et al. Characterization and antimicrobial properties of essential oils from four wild taxa of Lamiaceae family growing in Apulia. Agronomy 11, 1431. https://doi.org/10.3390/agronomy11071431 (2021).
Ma, L. & Yao, L. Antiviral effects of plant-derived essential oils and their components: An updated review. Molecules 25, 2627. https://doi.org/10.3390/molecules25112627 (2020).
Article CAS PubMed PubMed Central Google Scholar
Abd Rashed, A., Rathi, D.-N.G., Ahmad Nasir, N. A. & Abd Rahman, A. Z. Antifungal properties of essential oils and their compounds for application in skin fungal infections: Conventional and nonconventional approaches. Molecules 26, 1093. https://doi.org/10.3390/molecules26041093 (2021).
Article CAS PubMed PubMed Central Google Scholar
Haro-González, J. N., Castillo-Herrera, G. A., Martínez-Velázquez, M. & Espinosa-Andrews, H. Clove essential oil (Syzygium aromaticum L. myrtaceae): Extraction, chemical composition, food applications, and essential bioactivity for human health. Molecules 26, 6387. https://doi.org/10.3390/molecules26216387 (2021).
Article CAS PubMed PubMed Central Google Scholar
Salem, M. Z. M. et al. Plants-derived bioactives: Novel utilization as antimicrobial, antioxidant and phytoreducing agents for the biosynthesis of metallic nanoparticles. Microb. Pathog. 158, 105107. https://doi.org/10.1016/j.micpath.2021.105107 (2021).
Article CAS PubMed Google Scholar
Sartorelli, P., Marquioreto, A. D., Amaral-Baroli, A., Lima, M. E. L. & Moreno, P. R. H. Chemical composition and antimicrobial activity of the essential oils from two species of Eucalyptus. Phytother. Res. 21, 231–233. https://doi.org/10.1002/ptr.2051 (2007).
Article CAS PubMed Google Scholar
Gyawali, R. & Ibrahim, S. A. Natural products as antimicrobial agents. Food Control 46, 412–429. https://doi.org/10.1016/j.foodcont.2014.05.047 (2014).
Abdelsalam, N. R. et al. Morphological, biochemical, molecular, and oil toxicity properties of Taxodium trees from different locations. Ind. Crops Products 139, 111515. https://doi.org/10.1016/j.indcrop.2019.111515 (2019).
Iordache, O. et al. Antimicrobial activity of textiles treated with rosemary and orange essential oils against a selection of pathogenic fungi. Sci. Bull. Ser. F. Biotechnol. 20, 362–369 (2016).
Berechet, M. D., Chirilă, C. & Deselnicu, V. International Conference on Advanced Materials and Systems (ICAMS). 197–202 (The National Research & Development Institute for Textiles and Leather-INCDTP).
Mohamed, A. A. et al. Phytochemical compounds of branches from P. halepensis oily liquid extract and S. terebinthifolius essential oil and their potential antifungal activity. Processes 8, 330. https://doi.org/10.3390/pr8030330 (2020).
Gemperline, E., Keller, C. & Li, L. Mass spectrometry in plant-omics. Anal. Chem. 88, 3422–3434. https://doi.org/10.1021/acs.analchem.5b02938 (2016).
Article CAS PubMed Google Scholar
Abd El-Kareem , MS , Rabbih , M. & Selim , E. Semi-empirical and HOMO, LUMO studies of some chlorinated pesticide compounds .Commun.Computing.Chem.6, 1–12.https://doi.org/10.4208/cicc.2018.v6.n1.1 (2018).
Ali, H. M. et al. New approach for using of Mentha longifolia L. and Citrus reticulata L. essential oils as wood-biofungicides: GC-MS, SEM, and MNDO quantum chemical studies. Materials (Basel) https://doi.org/10.3390/ma14061361 (2021).
Article PubMed PubMed Central Google Scholar
Behiry, S. I. et al. Mass spectroscopic analysis, MNDO quantum chemical studies and antifungal activity of essential and recovered oil constituents of lemon-scented gum against three common molds. Processes 8, 275. https://doi.org/10.3390/pr8030275 (2020).
Taha, A. S. et al. Assessment of the impact of different treatments on the technological and antifungal properties of papyrus (Cyperus papyrus L.) sheets. Materials 12, 620. https://doi.org/10.3390/ma12040620 (2019).
Article MathSciNet CAS PubMed PubMed Central ADS Google Scholar
Abo Elgat, WAA et al.Eucalyptus camaldulensis, Citrus aurantium, and Citrus sinensis essential oils as antifungal activity against Aspergillus flavus, Aspergillus niger, Aspergillus terreus, and Fusarium culmorum.Proceedings 8, 1003. https://doi.org/10.3390/pr8081003 (2020).
Taha, A. S. et al. Impact of some plant source additives on enhancing the properties and antifungal activities of pulp made from linen fibers. Bioresources 14, 6025–6046. https://doi.org/10.15376/biores.14.3.6025-6046 (2019).
Nedorostova, L., Kloucek, P., Kokoska, L., Stolcova, M. & Pulkrabek, J. Antimicrobial properties of selected essential oils in vapour phase against foodborne bacteria. Food Control. 20, 157–160. https://doi.org/10.1016/j.foodcont.2008.03.007 (2009).
Salem, M. Z. M., Zidan, Y. E., Mansour, M. M. A., El Hadidi, N. M. N. & Abo-Elgat, W. A. A. Antifungal activities of two essential oils used in the treatment of three commercial woods deteriorated by five common mold fungi. Int. Biodeterior. Biodegrad. 106, 88–96. https://doi.org/10.1016/j.ibiod.2015.10.010 (2016).
Praveena, R., Prasath, D., Santhosh, JE & Anandaraj, M. (ICAR-IISR, 2018).
Bajpai, V. K., Shukla, S. & Kang, S. C. Chemical composition and antifungal activity of essential oil and various extract of Silene armeria L.. Bioresour. Technol. 99, 8903–8908. https://doi.org/10.1016/j.biortech.2008.04.060 (2008).
Article CAS PubMed Google Scholar
Rast-Eicher, A. Microscopy of Archaeological Textiles and Furs.16–18 (Budapest: Archaeolingua Foundation, 2016).
Sparnins, E. Mechanical properties of flax fibers and their composites, (2009).
Popescu, C. & Wortmann, F.-J. Wool–structure, mechanical properties and technical products based on animal fibres. Ind. Appl. Nat. Fibres Struct. Prop. Tech. Appl. 10, 255. https://doi.org/10.1002/9780470660324.ch12 (2010).
Bunsell, A. R. Handbook of Properties of Textile and Technical Fibres (Woodhead Publishing, 2018).
Wang, H., Siddiqui, M. Q. & Memon, H. In Cotton Science and Processing Technology: Gene, Ginning, Garment and Green Recycling (eds Wang, H. & Memon, H.) 79–97 (Springer, 2020).
Babu, K. M. (eds Ryszard M. Kozłowski & Maria Mackiewicz-Talarczyk) (Woodhead Publishing, 2020).
McLafferty, F. & Turecek, F. Interpretation of Mass Spectra. University Science Books (Mill Valley, 1993).
Mano, N. & Goto, J. Biomedical and biological mass spectrometry. Anal. Sci. 19, 3–14. https://doi.org/10.2116/analsci.19.3 (2003).
Article CAS PubMed Google Scholar
Dias, D. A. et al. Current and future perspectives on the structural identification of small molecules in biological systems. Metabolites 6, 46. https://doi.org/10.3390/metabo6040046 (2016).
Article CAS PubMed PubMed Central Google Scholar
Neumann, S. & Böcker, S. Computational mass spectrometry for metabolomics: Identification of metabolites and small molecules. Anal. Bioanal. Chem. 398, 2779–2788. https://doi.org/10.1007/s00216-010-4142-5 (2010).
Article CAS PubMed Google Scholar
Joshi, B. D., Mishra, R., Tandon, P., Oliveira, A. C. & Ayala, A. P. Quantum chemical studies of structural, vibrational, NBO and hyperpolarizability of ondansetron hydrochloride. J. Mol. Struct. 1058, 31–40. https://doi.org/10.1016/j.molstruc.2013.10.062 (2014).
Article CAS ADS Google Scholar
Muñoz-Caro, C., Niño, A., Senent, M. L., Leal, J. M. & Ibeas, S. Modeling of protonation processes in acetohydroxamic acid. J. Org. Chem. 65, 405–410. https://doi.org/10.1021/jo991251x (2000).
Article CAS PubMed Google Scholar
Akin, D. E., Rigsby, L. L., Henriksson, G. & Eriksson, K. E. L. Structural effects on flax stems of three potential retting fungi. Text. Res. J. 68, 515–519. https://doi.org/10.1177/004051759806800708 (1998).
Guckert, D. J., Lovasic, S. L. & Parry, R. F. Fibers and fabrics. In Handbook of building materials for fire protection, 89 (2004).
Taha, A. S. et al. Using plant extractives as eco-friendly pulp additives: Mechanical and antifungal properties of paper sheets made from linen fibers. BioResources 16, 2589–2606. https://doi.org/10.15376/biores.16.2.2589-2606 (2021).
Salem, M. Z., Abo Elgat, W. A., Taha, A. S., Fares, Y. G. & Ali, H. M. Impact of three natural oily extracts as pulp additives on the mechanical, optical, and antifungal properties of paper sheets made from Eucalyptus camaldulensis and Meryta sinclairii wood branches. Materials 13, 1292. https://doi.org/10.3390/ma13061292 (2020).
Article CAS PubMed PubMed Central ADS Google Scholar
Crawford, B. et al. Effect of fungal deterioration on physical and mechanical properties of hemp and flax natural fiber composites. Materials 10, 1252. https://doi.org/10.3390/ma10111252 (2017).
Article CAS PubMed PubMed Central ADS Google Scholar
Li, X., Tabil, L. G. & Panigrahi, S. Chemical treatments of natural fiber for use in natural fiber-reinforced composites: A review. J. Polym. Environ. 15, 25–33. https://doi.org/10.1007/s10924-006-0042-3 (2007).
McMullin, D. R., Sumarah, M. W. & Miller, J. D. Chaetoglobosins and azaphilones produced by Canadian strains of Chaetomium globosum isolated from the indoor environment. Mycotoxin Res. 29, 47–54. https://doi.org/10.1007/s12550-012-0144-9 (2013).
Article CAS PubMed Google Scholar
Pekhtasheva, EL, Neverov, AN, Kubica, S. & Zaikov, GE Biodegradation and biodeterioration of some natural polymers.Polym.Res.J. 6, 263–280 (2011).
Machnowski, W., Gutarowska, B., Perkowski, J. & Wrzosek, H. Effects of gamma radiation on the mechanical properties of and susceptibility to biodegradation of natural fibers. Text. Res. J. 83, 44–55. https://doi.org/10.1177/0040517512449045 (2012).
Kavkler, K., Gunde-Cimerman, N., Zalar, P. & Demšar, A. Fungal contamination of textile objects preserved in Slovene museums and religious institutions. Int. Biodeterior. Biodegrad. 97, 51–59. https://doi.org/10.1016/j.ibiod.2014.09.020 (2015).
Gakuubi, M. M., Maina, A. W. & Wagacha, J. M. Antifungal activity of essential oil of Eucalyptus camaldulensis Dehnh. against selected Fusarium spp.. Int. J. Microbiol. 2017, 8761610. https://doi.org/10.1155/2017/8761610 (2017).
Article CAS PubMed PubMed Central Google Scholar
Siramon, P., Ohtani, Y. & Ichiura, H. Chemical composition and antifungal property of Eucalyptus camaldulensis leaf oils from Thailand. Records Nat. Prod. 7, 49–53 (2013).
Su, Y.-C., Ho, C.-L., Wang, E.-C. & Chang, S.-T. Antifungal activities and chemical compositions of essential oils from leaves of four eucalypts. Taiwan J. For. Sci. 21, 49–61 (2006).
Barra, A., Coroneo, V., Dessi, S., Cabras, P. & Angioni, A. Chemical variability, antifungal and antioxidant activity of Eucalyptus camaldulensis essential oil from Sardinia. Nat. Prod. Commun. 5, 329–335 (2010).
Ez-Zriouli, R. et al. Chemical composition of essential oil of Eucalyptus camaldulensis collected from forest Moroccan and determination their antifungal activity on two phytopathogenic fungi. Plant Cell Biotechnol. Mol. Biol 20, 770–777 (2019).
Nikbakht, M. R. et al. The chemical composition and in vitro antifungal activities of essential oils of five Eucalyptus species. J. Essent. Oil Bear. Plants 18, 666–677. https://doi.org/10.1080/0972060X.2014.935061 (2015).
Elaissi, A. et al. Antibacterial activity and chemical composition of 20 Eucalyptus species’ essential oils. Food Chem. 129, 1427–1434. https://doi.org/10.1016/j.foodchem.2011.05.100 (2011).
Dogan, G., Kara, N., Bagci, E. & Gur, S. Chemical composition and biological activities of leaf and fruit essential oils from Eucalyptus camaldulensis.Journal for Natural Research C 72, 483–489.https://doi.org/10.1515/znc-2016-0033 (2017).
Bowers, J. H. & Locke, J. C. Effect of botanical extracts on the population density of Fusarium oxysporum in soil and control of fusarium wilt in the greenhouse. Plant Dis. 84, 300–305. https://doi.org/10.1094/pdis.2000.84.3.300 (2000).
Inouye, S. et al. Inhibitory effect of essential oils on apical growth of Aspergillus fumigatus by vapour contact. Mycoses 43, 17–23. https://doi.org/10.1046/j.1439-0507.2000.00538.x (2000).
Article CAS PubMed Google Scholar
Angelini, P., Pagiotti, R., Menghini, A. & Vianello, B. Antimicrobial activities of various essential oils against foodborne pathogenic or spoilage moulds. Ann. Microbiol. 56, 65–69. https://doi.org/10.1007/BF03174972 (2006).
Terzi, V. et al. In vitro antifungal activity of the tea tree (Melaleuca alternifolia) essential oil and its major components against plant pathogens. Lett. Appl. Microbiol. 44, 613–618. https://doi.org/10.1111/j.1472-765X.2007.02128.x (2007).
Article CAS PubMed Google Scholar
McLaren, R., Joseph, F., Baguley, C. & Taylor, D. A review of e-textiles in neurological rehabilitation: How close are we?. J. NeuroEng. Rehabil. 13, 59. https://doi.org/10.1186/s12984-016-0167-0 (2016).
Article PubMed PubMed Central Google Scholar
Martel, B., Campagne, C. & Behary-Massika, N. When textiles heal you.Med.Sci.(Paris) 33, 73–80 (2017).
Walentowska, J. & Foksowicz-Flaczyk, J. Thyme essential oil for antimicrobial protection of natural textiles. Int. Biodeterior. Biodegrad. 84, 407–411. https://doi.org/10.1016/j.ibiod.2012.06.028 (2013).
Kriangkraipipat, T., Wittayakunsathit, N., Ngamsritrakul, T. & Panyametheekul, S. Efficiency on fungal inhibition of benzalkonium chloride and lemongrass essential oil on polyester fabric. Eng. J. 24, 23–33. https://doi.org/10.4186/ej.2020.24.1.23 (2020).
Golja, B. & Tavčer, P. F. Textile functionalisation by printing fragrant, antimicrobial and flame-retardant microcapsules. Tekstilec 59, 278–288 (2016).
Yang, Z. M. et al. Advanced Materials Research. 308–315 (Trans Tech Publ).
Liu, J. et al. Study on the grafting of chitosan–gelatin microcapsules onto cotton fabrics and its antibacterial effect. Colloids Surf. B Biointerfaces 109, 103–108. https://doi.org/10.1016/j.colsurfb.2013.03.040 (2013).
Article CAS PubMed Google Scholar
He, G. R. et al. Materials science forum. 2281–2284 (Trans Tech Publ).
Li, L., Song, L., Hua, T., Au, W. M. & Wong, K. S. Characteristics of weaving parameters in microcapsule fabrics and their influence on loading capability. Text. Res. J. 83, 113–121 (2013).
Muresan, A., Cerempei, A., Dunca, S., Muresan, R. & Butnaru, R. Aromatherapeutic characteristics of cotton fabrics treated with rosemary essential oil. Cellul. Chem. Technol. 43, 435–442 (2009).
Matusiak, K. et al. Application of Cinnamomum zeylanicum essential oil in vapour phase for heritage textiles disinfection. Int. Biodeterior. Biodegrad. 131, 88–96. https://doi.org/10.1016/j.ibiod.2017.02.011 (2018).
Morcia, C., Malnati, M. & Terzi, V. In vitro antifungal activity of terpinen-4-ol, eugenol, carvone, 1,8-cineole (eucalyptol) and thymol against mycotoxigenic plant pathogens. Food Addit. Contam. Part A 29, 415–422. https://doi.org/10.1080/19440049.2011.643458 (2012).
Oxenham, S. K., Svoboda, K. P. & Walters, D. R. Antifungal activity of the essential oil of Basil (Ocimum basilicum). J. Phytopathol. 153, 174–180. https://doi.org/10.1111/j.1439-0434.2005.00952.x (2005).
Rahmouni, A. et al. Chemical composition and antifungal activity of five essential oils and their major components against Fusarium oxysporum f. sp. albedinis of Moroccan palm tree. Euro-Mediterr. J. Environ. Integr. 4, 27. https://doi.org/10.1007/s41207-019-0117-x (2019).
Al-Ja’fari, A.-H. et al. Composition and antifungal activity of the essential oil from the rhizome and roots of Ferula hermonis. Phytochemistry 72, 1406–1413. https://doi.org/10.1016/j.phytochem.2011.04.013 (2011).
Article CAS PubMed Google Scholar
do Nascimento, K. F. et al. Antioxidant, anti-inflammatory, antiproliferative and antimycobacterial activities of the essential oil of Psidium guineense Sw. and spathulenol. J. Ethnopharmacol. 210, 351–358. https://doi.org/10.1016/j.jep.2017.08.030 (2018).
Article CAS PubMed Google Scholar
Open access funding provided by The Science, Technology & Innovation Funding Authority (STDF) in cooperation with The Egyptian Knowledge Bank (EKB).
Conservation Department, Faculty of Archaeology, Aswan University, Aswan, 81528, Egypt
Restoration Department, High Institute of Tourism, Hotel Management and Restoration, Abu Qir, Alexandria, Egypt
Ibrahim HM Ibrahim & Wael AA Abo-Elgat
Department of Plant Protection, Faculty of Agriculture (Saba Basha), Alexandria University, Alexandria, 21531, Egypt
Forestry and Wood Technology Department, Faculty of Agriculture (EL-Shatby), Alexandria University, Alexandria, 21545, Egypt
Atomic and Molecular Physics Unit, Experimental Nuclear Physics Department, Nuclear Research Centre, Egyptian Atomic Energy Authority, Inshas, Cairo, 13759, Egypt
You can also search for this author in PubMed Google Scholar
You can also search for this author in PubMed Google Scholar
You can also search for this author in PubMed Google Scholar
You can also search for this author in PubMed Google Scholar
You can also search for this author in PubMed Google Scholar
You can also search for this author in PubMed Google Scholar
All authors have equal contributions in methodology, analysis, collecting and writing the article.
Correspondence to Mohamed Z. M. Salem.
The authors declare no competing interests.
Springer Nature remains neutral with regard to jurisdictional claims in published maps and institutional affiliations.
Open Access This article is licensed under a Creative Commons Attribution 4.0 International License, which permits use, sharing, adaptation, distribution and reproduction in any medium or format, as long as you give appropriate credit to the original author(s) and the source, provide a link to the Creative Commons licence, and indicate if changes were made. The images or other third party material in this article are included in the article's Creative Commons licence, unless indicated otherwise in a credit line to the material. If material is not included in the article's Creative Commons licence and your intended use is not permitted by statutory regulation or exceeds the permitted use, you will need to obtain permission directly from the copyright holder. To view a copy of this licence, visit http://creativecommons.org/licenses/by/4.0/.
Taha, A.S., Ibrahim, I.H.M., Abo-Elgat, W.A.A. et al. GC–MS, quantum mechanics calculation and the antifungal activity of river red gum essential oil when applied to four natural textiles. Sci Rep 13, 18214 (2023). https://doi.org/10.1038/s41598-023-45480-x
DOI: https://doi.org/10.1038/s41598-023-45480-x
Anyone you share the following link with will be able to read this content:
Sorry, a shareable link is not currently available for this article.
Provided by the Springer Nature SharedIt content-sharing initiative
By submitting a comment you agree to abide by our Terms and Community Guidelines. If you find something abusive or that does not comply with our terms or guidelines please flag it as inappropriate.

Corduroy Fabric Scientific Reports (Sci Rep) ISSN 2045-2322 (online)